Motivation and overview
What are the general principles that govern the emergence of life-enabling behaviour from a collection of (dead) molecules? How can we understand the emergence of a given behaviour, such that we can design interventions to modify it, for example to cure a disease? These are the overarching questions that drive our research. In order to address them, we study fundamental cellular regulatory processes, mainly signal transduction and gene regulation, using theory and mathematical models, coupled with the analysis of experimental data from collaborating groups.
Life emerges from the dynamical interactions among many components at the molecular, cellular and supra-cellular levels. Therefore, when we study a given process we can never account for all the elements involved in it with the same level of detail. Typically, we choose a subset of components to focus on (“system”), and abstract away the rest into the so-called “context”. However, the context and the system are coupled. The behaviour of a given system will typically depend on the context, but how and why is often not well understood. We are particularly interested in this "context problem". In addition to investigating qualitative effects of the context brought by the presence of different molecular components, we are particularly interested in quantitative effects, brought by differences in concentrations or reaction rates. Beyond revealing fundamental biological insight, we ultimately hope this research will facilitate practical progress in applied areas from synthetic biology to personalized medicine, where for example the behaviour of a gene circuit depends on the cell type, and two different people provide two different contexts for the effects of mutations or drugs.
![rosa_illustration[1].png](https://static.wixstatic.com/media/dd1693_26216e56119f4a5fb9457739e765ad9d~mv2.png/v1/fill/w_509,h_327,al_c,q_85,usm_0.66_1.00_0.01,enc_avif,quality_auto/rosa_illustration%5B1%5D.png)
When we study a given process, we must always choose a subset of elements to focus on (“system”). The rest is left into the “context”. Different contexts can lead to fundamentally different behaviours. We are interested in revealing general principles underlying context-dependency.
Current research
Eukaryotic gene regulation
Controlling which genes are expressed in a cell and to what levels at any particular time is the most fundamental layer of cellular regulation. This highly controlled process is orchestrated by proteins called transcription factors, which are able to recognise specific sites on the DNA, and by interacting with other proteins and the transcriptional machinery, increase or decrease the transcription rate of genes. In eukaryotic genomes, any given gene is regulated by many transcription factors that act in combination. We are studying the principles that govern such combinatorial control of transcription, and are particularly interested in the interplay between transcription factors and chromatin dynamics. I started this work focusing on biophysical models of gene regulation based on the "linear framework" formalism developed by the Gunawardena group, and we have recently started investigating how to combine these mechanistic models with deep learning models in order to extract knowledge from high-throughput genomic datasets. We are currently collaborating with the Velten group (CRG), Zeitlinger group (Stowers) and Lenstra group (NKI) on this topic.
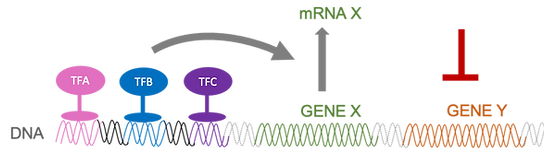
Related publications:
Emergence of activation or repression in transcriptional control under a fixed molecular context.
Synthetic enhancers reveal design principles of cell state specific regulatory elements in hematopoiesis.
The Hill function is the universal Hopfield barrier for sharpness of input-output responses.
Allosteric conformational ensembles have unlimited capacity for integrating information.
See also the related talks.
Alternative splicing regulation
Eukaryotic genes typically contain exons and introns, with the introns removed and exons joined together in the final mRNA products. Given that exons can be joined in multiple alternative patterns, this enables the generation of many proteins from a single gene, and thus an expansion of proteomic diversity without having to increase the number of genes. At the same time, this involves careful regulation of which isoforms are produced, and disregulation of this process is relevant to multiple diseases. Relatively little is known about how the splicing machinery interacts with splciing regulators and the mRNA sequences from a quantitative and dynamical perspective. We aim to contribute to this problem through developing mechanistic models as well as hybrid mechanistic-deep learning models. We are currently collaborating with the Valcárcel group (CRG) on this topic.
Related publications:
Past research
Learning in single cells
Single cells have an extraordinary capacity to sense and respond to stimuli, but they are typically not considered to be capable of learning. Yet, there have been experimental reports in this direction, and modern experiments are confirming that single cells are capable of learning behaviours. We developed a model for how one of the most basic forms of learning, habituation, could arise from common networks of signalling proteins inside a cell.
Pulsing in signalling circuits
Many cellular regulatory proteins, like the well-known mammalian kinases ERK and p38, and the transcription factors p53 or NF-kB, exhibit pulses of concentration or activity over time, which can have a defined periodicity or not (stochastic). These pulsatile dynamics can encode the identity and intensity of activating inputs, so that different dynamics can mediate different downstream responses. I studied mechanisms for both pulse generation and decoding.
Related publications:
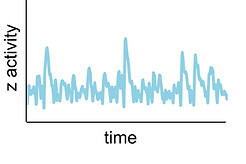
Oscillations in Bacillus subtilis biofilms
Despite bacteria are unicellular organisms, they are often found in multicellular aggregates known as biofilms. The group of Prof. Gürol Süel discovered that in biofilms of B. subtilis, the bacteria can communicate via potassium-mediated electrical signalling. Moreover, when growing biofilms reach a certain size, their growth starts to periodically stop, and this is accompained by population-level oscillations in glutamate metabolism and potassium-mediated electrical signalling. I worked with them to develop models at various levels of abstraction to characterise these oscillations and understand their properties.
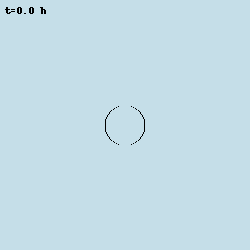
Model simulation of an oscillating biofilm.
Related publications:
Cell-cell contact area as a modulator of signalling and cell fate
The activity of transcription factors and thus gene regulation depend on intracellular and extracellular signals. In order to enable communication between cells and their environment, many signalling pathways have evolved. One such pathway is the Notch signalling pathway. In this case, the receptor on a cell is activated by a transmembrane ligand on neighboring cells. Commonly, one of the consequences of Notch activation is the repression of the expression of the pathway ligand Delta. As a result, neighboring cells signalling through Notch-Delta often adopt different fates. We studied under what conditions this same mechanism would mediate symmetric fates, and found a potential link between cell-cell contact area and signalling using Drosophila intestinal stem cells as an experimental model.

Cartoon showing that higher contact area promotes the activation of Notch signalling and stem cell differentiation. From Guisoni, Martinez-Corral et al., Development, 2017.